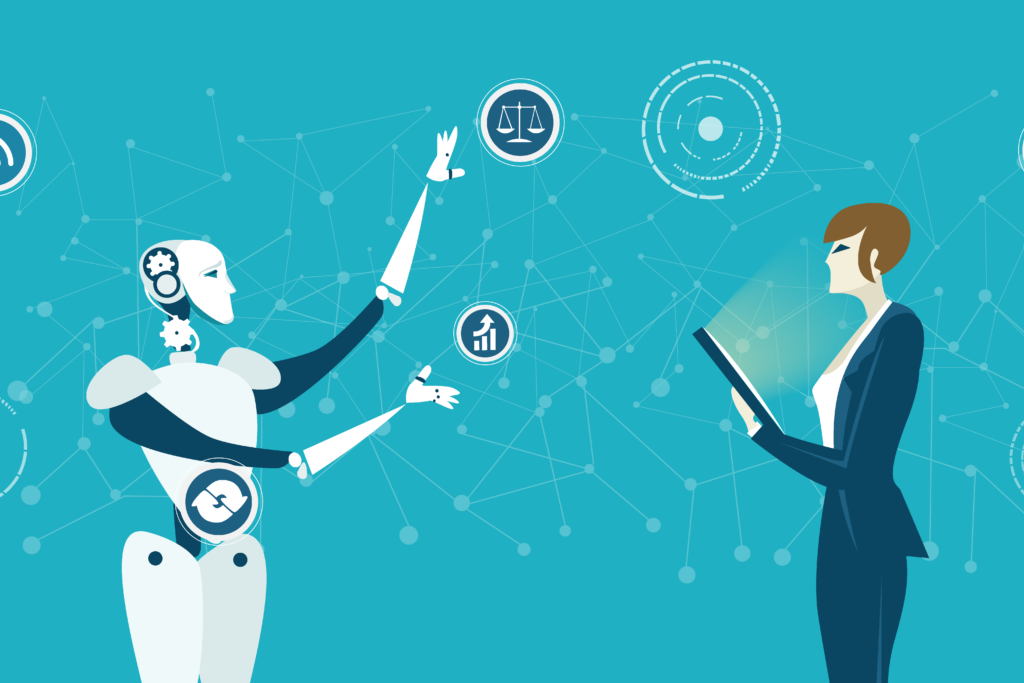
What is a cyborg? When hearing this word, most people think of science fiction. They picture the replicants from Blade Runner or Darth Vader from Star Wars. However, in its purest definition, cyborgs already exist.
After all, the use of technology in the medical field is something that is already saving millions of lives and allowing people to live with a quality of life they would otherwise never be able to achieve. And, if cyborgs – cybernetic organisms – are defined as creatures that are part-human and part-machine, what would you call a person with a pacemaker?
But, sometimes, technology catches up to fiction. Take, for example, Neuralink’s recent announcement, in which Elon Musk’s neurotechnology company unveiled they’d implanted a coin-sized computer chip into the brain of a pig called Gertrude. Musk sees this as the first step in the development of brain-machine interfaces (BMI) and, eventually, human-AI symbiosis.
Although the time when humans can fully merge with AIs to become the cyborgs we see in the movies is still years of testing and research away, academics have been studying for decades the medical applications of AI and currently use it to create tools that improve patients’ quality of life.
Granted, we might have never met a cyborg, but we all have heard of Stephen Hawking.
Lama Nachman, Intel Fellow and Director of Intel’s Anticipatory Computing Lab, was among the team of scientists that helped Hawking speak. When he was 21 years old, Hawking was diagnosed with a type of motor neurone disease called amyotrophic lateral sclerosis (ALS). However, during a visit to CERN in 1985, Hawking contracted pneumonia which, combined with ALS, was life-threatening. To save his life, the doctors performed a tracheotomy, a procedure that cost him his ability to speak.
From 2012, Nachman’s team developed the Assistive Context-Aware Toolkit (ACAT), a software that allowed Hawking to communicate using keyboard simulation, word prediction and speech synthesis. Hawking had a sensor placed on his glasses. When the scientist moved his cheek, he triggered the sensor and could interface with his computer.
After Hawking’s death in 2018, Intel made ACAT open-source, allowing other people with ALS, or any other disability, to use it or even develop it further. This decision was a fundamental step in democratising the technology, a vision that Hawking shared.
“Even in the early conversations with Stephen, he was adamant about ‘if you guys want me to spend a lot of effort and energy to work with you on this, it needs to be something that is open-source,” Nachman tells Tech For Good.
Now, almost 10 years later, Nachman is working with another world-famous researcher, Dr Peter Scott-Morgan, who also suffers from ALS. Scott-Morgan recently underwent the same surgery that made Hawking lose his ability to speak. However, unlike Hawking, Scott-Morgan is determined to fight the progress of his disease by replacing his organs with pieces of machinery. He has said he wants to become “the world’s first full cyborg”.
Working alongside Scott-Morgan, Nachman and her team are looking to integrate gaze-tracking and AI into ACAT. Nachman’s aim is to develop a response-generation capability that listens to Scott-Morgan’s conversations and suggests possible responses. This feature would allow users to maintain the speed of a standard conversation, something that is proving, at the moment, quite challenging.
“The gap is always the same,” Nachman explains. “Even if you predicted what they’re going to say before they finish the sentence, there’s still quite a bit of delay before you can actually formulate your response and send it which, for someone who’s many, many, many days at the other end of that conversation, it’s a really hard thing to do. It just breaks that connection.”
Reminiscing on her experience working with Hawking, Nachman details his desire to understand and control the technology. In contrast, Scott-Morgan is happy to let go of control. “Peter was literally at the opposite end of the spectrum,” she says. “He was like ‘I am a cyborg. Peter 2.0 is not about me wanting to control the AI, it’s about me and the AI becoming what Peter 2.0 is’. So he had a very open nature to all of that.”
Nachman believes that context-aware computing and AI-human collaboration technologies can improve many people’s lives, as long as they can be developed and used responsibly.
“One of the things that has happened over the last four or five years is that the public perception of AI has gone downhill dramatically,” she says. “And I think a big part of that has been that we have a gap in responsibility. I think it’s really up to all of us as AI researchers to make sure that we’re developing responsible technology. We can’t just put things out in the world, not consider the ramifications of what these things mean, and hope for the best.”
Over the last four or five years the public perception of AI has gone downhill dramatically
And, ‘the best’ is indeed very promising. Besides the addition of AI, Nachman’s team also wants to add a brain computer interface (BCI) into the software, using electroencephalograms (EEGs). EEGs are commonly used tests that use electrodes to track and record brain waves. Nachman envisions skullcaps that would use EEG technology to interface with a computer, similarly to Neuralink’s chips. This would make ACAT accessible to those who can’t move any muscle of their bodies to trigger a sensor. For these people, their brain waves would trigger speech.
Nachman’s commitment to this project is rooted in her long experience working with people with ALS. “It’s kind of the first thing that you hear from them,” she says. “‘I just want to be able to communicate. I want to be able to communicate with my love. I want to be able to express a thought’. And that is really what humanity is all about.”
Allowing people to communicate is also the aim for Dr Rylie Green. Her research has greatly improved the quality of cochlear implants and bionic eyes, helping people to hear and see.
Green is a Reader in Biomedical Engineering at Imperial College London, where she specialises in researching bioactive conducting polymers; that is, materials that can be used in medical devices.
Green explains that the main reason why implantable devices are only rarely commercialised is because of the difficulty of connecting living body tissue with metals. “There are only a very few numbers of electrodes that you can actually fit within these tissues and safely use,” she tells Tech For Good.
This is because of two reasons. Firstly, living systems communicate with ions, not electrons. Injecting electrical currents into the body often causes dangerous chemical reactions. To solve this problem, Green’s team is researching materials that convert electrodes’ electrical charge to an ionic charge that the body understands. If she succeeds, electronic devices would become safer to use and able to communicate with any organ.
Secondly, the body rejects inorganic materials, like metals, and creates scar tissue around them. These scars are meant to protect the body from harmful elements but also prevent implantable devices from working.
Green wants to create electrodes with materials that are “soft and squishy”, like the body’s nervous tissue. To achieve this, her team uses hydrogels, materials that are very compatible with the body. These should be very familiar, as they are used to make contact lenses.
But, why imbed metals when you can imbed living organisms?
The next step of Green’s research is what she calls “living electrodes,” a combination of hydrogels and STEM cells that create connections between electrodes and body tissue.
“Most of the tissues that we’re trying to interface with have some sort of disease state,” she says. “It might be blindness caused by loss of cells in the eye, deafness or things like Parkinson’s, dementia, where you’re getting abnormalities within brain activity. All of those cells are in a state of stress. Behind this project is the idea that, if we provide healthy cells in this environment, and instead of stimulating electrically, we give them a natural ionic input, we might create better results for patients because we’re creating an interface that’s very natural, instead of an artificial electrical stimulation that can also continue to cause some damage.”
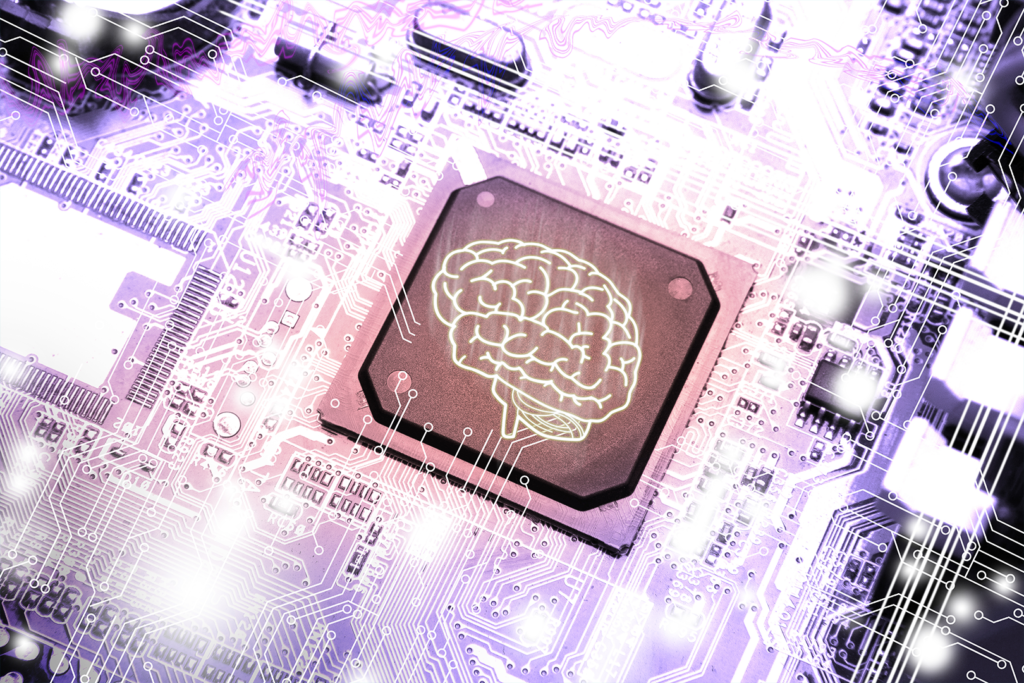
Although the project is promising, it comes with many challenges. The most pressing one is how to support the growth of the STEM cells and make sure that they develop the correct characteristics and connections to be useful to the device.
The types of projects that Green has worked on alongside companies such as Cochlea Ltd., Bionic Vision Technologies and Galvani Bioelectronics are based on a very similar concept to the one used by Neuralink. While Green doesn’t believe that Elon Musk’s company is doing anything particularly revolutionary, she underlines the importance of his investment in this technology.
“Academics and industry partners have been working with these technologies for decades,” she says. “But this is what we’ve needed to push this industry for a long time: for somebody to put their money where their mouth is, and to drive the industry to make new materials, miniaturised devices and new chip technologies, bring them all together and show that they can work to actually push them into the market.”
As an example, Green talks about Cochlear, a cochlear implant company that she has worked with. Despite the developments that her team has made to increase the number of electrodes in cochlear implants, improving the user’s ability to differentiate sounds, the number of electrodes currently used is still the same as it was in the 1970s.
“Companies like Cochlear haven’t been driven yet to increase the number of electrodes,” she says. Musk’s investment into this type of technology could be the step necessary to drive this field forward; the one that other companies have not been willing – or able – to make.
Green believes that investing time and money into AI-human collaboration research will drastically change the lives of people that live with chronic diseases. A great example is epilepsy. This condition is poorly treated and poorly understood because, among other things, the focal point of seizures constantly changes. In Green’s best-case scenario, patients would have smart implants that used AI to predict an oncoming seizure and block it.
“Then, over time, you will have patients that can be walking around, operating heavy machinery, doing whatever they like, who may have normally been rather restricted or homebound, because they would have been more worried about having seizures,” she says. “I see that as being the best use of AI in implantable devices for humans.”
However, she admits that she’s not “quite seeing the cyborg army yet”. Creating extra functionality for human beings as Musk strives to do is, in her opinion, a long way away, mainly because of safety concerns. After all, any kind of implantable device is bound to a high-risk surgery and potential brain damage. This is the reason why surgeries such as deep brain stimulation procedures are done only on people who suffer serious conditions. Although this is a risk Scott-Morgan is willing to take, not many other people would.
If few people are willing to undergo brain surgery to become cyborgs, even fewer are willing to go through it more than once. But, surprise, here’s the catch: this is exactly what needs to happen in order to change the device’s battery.
To make Musk’s and Scott-Morgan’s dreams a reality, brain implants need to be safe, stable, and efficient. Moreover, as mentioned before, they need to be able to convert electricity into signals that the body can interpret. Only then will they become truly revolutionary.
We can't just put things out in the world, not consider the ramifications of what these things mean, and hope for the best
PEDOT might be the perfect solution.
PEDOT, poly(3,4-ethylenedioxythiophene) polystyrene sulfonate, is the material that both Green and Musk are using to try to overcome the challenges of implanting electrodes into the human brain. PEDOT was first discovered to have medical applications by Dr David Martin, currently a Professor and Associate Dean at the College of Engineering of the University of Delaware.
“It’s been an ongoing effort for more than 20 years now, really,” Martin tells Tech For Good when reflecting on the development of these polymers. The idea that he envisioned then, and that he is still pursuing, is to make coatings that lower the impedance of the electrodes that are implanted in the body, improving their biocompatibility.
Impedance is a huge challenge for makers of biomedical devices. Essentially, it is a circuit’s resistance to an alternating current. In the biomedical field, it determines the size and voltage that electrodes need to have. The only way to obtain small and long-lasting chips is to ensure that they can maintain a low impedance.
PEDOT was the first polymer that showed signs of being able to do just that. “What our materials do is they essentially create a lot of effective surface area,” Martin explains. “So the whole volume of the material is acting like a big open structure, and you can get a very efficient electron-to-ion charge exchange. Because of that, a little thin coating of our material will lower the importance of the electrode by 100 times, sometimes 1,000 times.”
However, a lowered impedance is not the only use of PEDOT. A big reason why these materials might be revolutionary is that they are good at conducting both electricity and ions, becoming the perfect bridge between implants and brain tissue. Over the last 20 years, Martin and his team have worked in testing and researching this property, as well as making new versions of EDOT (the monomer that originates PEDOT) that adapt to specific organs and devices.
“Hopefully we can come up with a big library of special source,” he says. “And then you can use whatever code you need based on, for example, whether the electrode you’re coding is made out of gold or stainless steel, or platinum. Or if you’re talking about electrodes that are going to go in the eye, or the brain or in the heart, then there are molecules that are specific to those kinds of tissues. And you would like molecules on your EDOT that are tailored to improve and optimise the interactions, stability, longevity, sensitivity… all the other properties that you might be interested to have in your device.”
PEDOT and EDOT still have a long way to go in terms of research stages. However, if they are proven successful, these materials could pave the way to make Scott-Morgan’s ‘Peter 2.0’ a reality.
Nonetheless, Martin argues that cyborgs are already around us. “At what point do we say the use of technology makes you a cyborg?” he says. “If you need a cane to walk, does that make you a cyborg? Or if you own a pair of shoes? A pair of shoes protects your feet against the ground and it’s not natural, we’re not born with shoes.”
Are cyborgs evil? It probably depends on your definition, but the common expert answer is ‘no’. Only humans are. “I think anything can be used for bad,” Nachman says. “But I am a huge optimist in terms of how technology can really come and amplify human potential.”
AI-human symbiosis has great potential for good, as well as great potential for bad; just like nuclear fission and genetic engineering. If the technology is perfected, regulated and democratised, like ACAT was, it could help Scott-Morgan speak and Elon Musk download his memories, but also allow millions of people hear, see, or live their lives without fear of having a seizure.
After all, most people don’t want to be super-humans; they just want to live a normal life.